“Normalcy is the antithesis of evolution.”
Siddhartha Mukherjee, The Gene: An Intimate History
Cancer is a disease we all know too well and a main reason why people die. Why is this? Cancer is not a new problem. In fact, it exists since thousands of years. It has been studied to unimaginable levels of detail. Clinicians are seeing the same disease over and over again. Then, why do people still die of cancer?
1. How things used to be
It’s 1938, and Public Health Services are advising people that detecting and treating cancers early will save their lives (Figure 1).
Now fast-forward nowadays. We hear the exact same core message from the Public Health Services of our times, gradually and consistently backed up by more and more data (Figure 2).
How and why is public health advice from 1938 so relevant today, 85 years later? Does this mean we made no progress in curing cancer?
Certainly not. Let me show you why.
2. What we accomplished
By zooming into cancer medical care in 1938, we quickly realize how rudimentary it actually was (Figure 3). Take radical mastectomy as an example: a truly invasive procedure developed by William Stewart Halsted at the end of the 19th century, in which the whole breast, all lymph nodes under the arm, and the chest wall muscle were removed. Radical mastectomies were still the norm following breast cancer diagnosis until 1970s. Nowadays, such surgery is almost never done, and has been replaced by local removal of the tumor (lumpectomy), followed or preceded by multiple options of additional therapies.
Now, let’s better understand the real impressive transformations that cancer science and medical practice have undergone in the last decades. I’ve chosen 10 such milestones (1938-2023) to emphasize below (Figure 4). This list is by absolutely no means exhaustive or even comprehensive, rather it is meant to contour the conceptual backbone of this progress (see here a more detailed list of cancer milestones).
1941 - Hormonal Therapy for Prostate Cancer. Charles Huggins discovers that lowering testosterone production by removing the testicles or by administering estrogen leads to shrinkage of prostate tumors. Hormonal therapy continues to be a main treatment for prostate cancer nowadays.
1958 - Combination Chemotherapy. James F. Holland, Emil Freireich, and Emil Frei propose that using multiple chemotherapy agents simultaneously will make it more difficult for tumors to become resistant. Their hypothesis builds upon generalizing the current state of art in tuberculosis treatment, in which the combination of antibiotics was more efficient than single agents. Nowadays, combination chemotherapy is a widespread standard of care in cancer centers around the world.
1978 - Tamoxifen. An anti-estrogen drug, originally developed as a birth control treatment, is approved by the FDA for the treatment of estrogen receptor positive (ER+) advanced breast cancer. In the following years, Tamoxifen will be shown to also be effective for early disease. Tamoxifen is a targeted treatment, i.e. it specifically targets only a particular type of cancerous cells (unlike chemotherapy). It is delivered as a tablet, and is currently the gold standard treatment for ER+ breast cancers, which make up the overwhelming majority (80%) of all newly-diagnosed breast cancers.
1998 - Trastuzumab. FDA approves Herceptin (Trastuzumab), a monoclonal antibody that inhibits the tumor-stimulating effects of the overexpressed amplicon HER2, for the treatment of metastatic HER2+ breast cancers (and later on also for treating early-stage HER2+ cases). Therapies involving Trastuzumab (usually in addition to chemotherapy) currently remain the gold standard in treating HER2+ breast cancers.
2001 - Imatinib. FDA approves Imatinib (Gleevec) for the treatment of chronic myeloid leukemia (CML), transforming a cancer death sentence into a manageable condition. Back in 1960s, Peter Nowell and David Hungerford (then a researcher at the University of Pennsylvania) found that CML patients had an abnormally short chromosome (later named the Philadelphia chromosome). Later on, Nora Heisterkamp at NCI identifies the reason why the Philadelphia chromosome was shorter: when this chromosome forms, two genes get fused together, forming the BCR-ABL fusion protein. Further, when this fusion happens in blood cells, it causes CML. The drug Gleevec, first conceptualized by Brian Drucker at Oregon University, blocks this fusion, hence only targeting faulty cells that display it. 5 years after the first phase 1 clinical trial of Imatinib (1998), 98% of treated CML patients were disease-free. Nowadays, Imatinib is considered a potential curative treatment: if a CML patient is in remission after 2 years of Imatinib treatment, their life expectancy is the same as a person who doesn’t have cancer. In May 2001, this monumental discovery makes it on the cover of TIME magazine (Figure 5).
2006 - Gardasil. On a completely different line of research, the first vaccine for the prevention of a human cancer is approved by the FDA. Gardasil, originally developed by researchers at the University of Queensland in Australia, protects against infections from four Human Papillomavirus (HPV) strains: HPV16 and 18, causing 70% of cervical cancers, and HPV 6 and 11, causing 90% of genital warts. Harald zur Hansen, who contributed to proving that genital HPV infections can causally lead to cervical cancer, is awarded the Nobel Prize in 2008 for this discovery. The approval of this vaccine marks an essential milestone also because it is a therapy for preventing cancer occurrence, rather than treating existing tumors. The current form of this vaccine is Guardasil 9, which protects against 5 additional HRV types.
2011 - Ipilimumab. In all fairness, the last decade belongs to immunotherapy, which aims to boost the natural body’s defense system to eliminate cancerous cells. Even though the idea of unleashing the immune system against cancer goes back hundreds of years, most substantial immunotherapy clinical advances have been very recent, facilitated by technological progress. Ipilimumab is the first FDA-approved immune checkpoint inhibitor, which are monoclonal antibodies that release the breaks of the immune system to trigger immune-mediated anti-tumor responses. Immune checkpoints themselves are molecules that work to maintain immune tolerance, but are often used by tumor cells to their advantage, to evade immunosurveillance. Ipilimumab activates the immune system by targeting CTLA-4, a co-inhibitory molecule expressed on T cells which negatively regulates T cell activation.
2014 - Pembrolizumab (Keytruda) is the first PD-1 immune checkpoint inhibitor approved by the FDA. PD-1 is expressed on the surface of several immune cell types (including T cells) and acts as a negative regulator of immune response. Its ligand PDL-1 is regularly expressed in normal tissues and regulates immune tolerance by suppressing T cell-mediated immune proliferation and cytokine secretion when binding with PD-1. The interaction between PD-1 and PDL-1 diminishes the activity of the immune system and plays an essential role in preventing autoimmune disease. But, tumor cells hijack this mechanism and abnormally express PDL-1 to escape immune surveillance. Antibodies targeting PD-1 or PDL-1 have been approved for the treatment of multiple cancer types. In 2017, a conceptual shift in cancer immunotherapy takes place, and Keytruda is approved for general use in any tumor with a certain genetic profile (based on tumor mutational burden), rather than a specific site. The blockage of the PD-1 pathway has been very successful in the clinic. Currently, most cancer types can benefit from this treatment.
2017 - Kymirah (Tisagenlecleucel) is approved by the FDA as the first chimeric antigen receptor (CAR) T cell therapy. CAR T-cells are T cells which are genetically modified and expanded ex vivo (outside the patient) to mount personalized tumor attacks, by targeting tumor-specific mutations displayed on the surface of cancer cells (called neoantigens). These engineered fighters are then infused back into the patient, with the idea of selectively attacking the cells they were designed to target. A pioneering early-stage study in late 2022 coupled non-viral CAR-T therapy with CRISPR editing, showing promise for increased clinical efficacy of CAR-T products. As of today, multiple cancer types have shown sustained and real clinical responses to immunotherapy, even though 3 main drawbacks still exist 1) immunotherapy is very expensive; 2) response rates remain limited; 3) there is no consistent satisfactory understanding of clinical or genetic factors predicting these responses.
2020 - AI in cancer. This decade belongs to artificial intelligence, which is quickly transforming the way we understand and interact with our own biology. Large AI models can now efficiently learn the image features separating cancerous lesions from normal ones from large amounts of labeled data. Such models are already deployed in medical centers across the world to assist with clinical cancer diagnosis. The pace of change is extremely rapid, and powerful AI models will soon be able to 1) assist in effective and efficient drug design; 2) accurately predict patient-specific personalized responses to various types of therapies; 3) flag high-risk conditions for clinical management, with the goal of preventing cancer from occurring in the first place. As more and more data is being produced and labeled, the applications and utility of such models in healthcare will skyrocket.
Ok, this all sounds truly impressive. But how much does it matter in the end? How do all these great therapeutic discoveries factor in for the one metric that we humans care about most: the time we have to live?
The harsh truth is that, despite great progress in cancer research, the drop in death rate from cancer is not as impressive. This becomes even more apparent when compared with what used to be the main source of death in the developed world (cardiovascular disease), for which the drop has been twice as large (Figure 6; I chose to display Switzerland as a best-case scenario for healthy lifestyle and optimal healthcare).
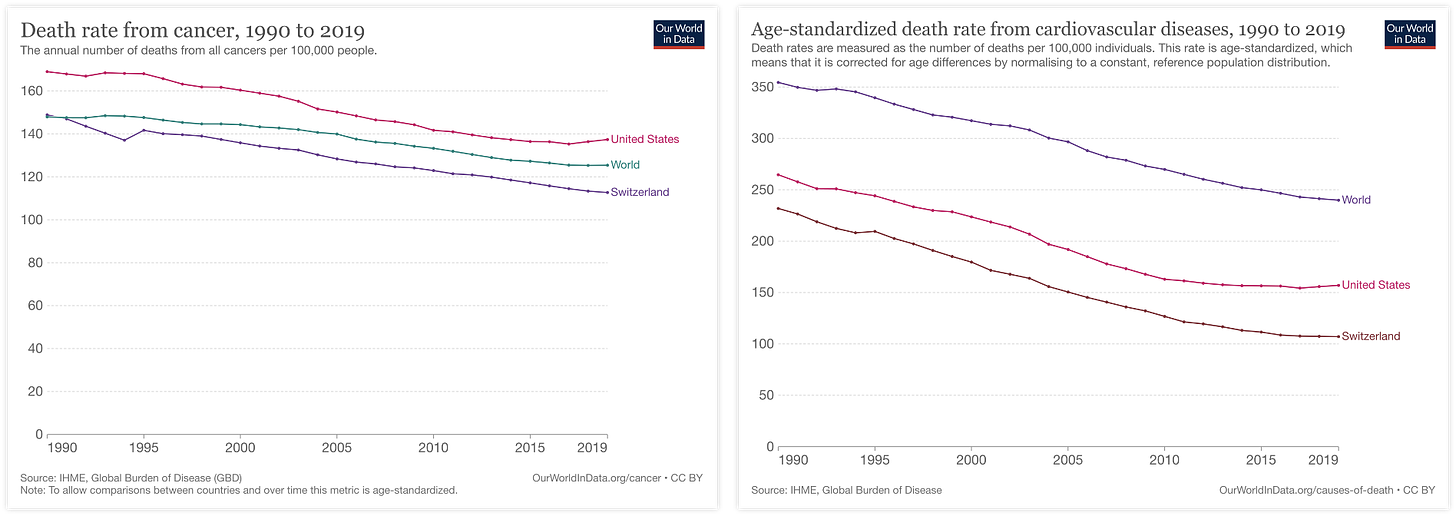
There are lots of complex reasons to explain the differences in magnitudes, some more intuitive than others. Nevertheless, it does leave us thinking. And it begs the question: why is progress against cancer so difficult?
3. Why cancer still wins
Cancer is a relentless evolutionary process. Cancer evolves to escape most therapies we use against it, no matter how potent. Every tumor is its own different word (Figure 7), and everything cancers want is to live. Fighting an evolutionary desire to live is hard. Cancer is a living process, but not a foreign one. It’s a living process living its own parallel life, as part of ours. Gradually, it hijacks and overtakes our own inner working mechanisms. This is why, conceptually, cancer is a very hard problem. Because, in a sense, the enemy is ourselves. It’s all different pieces of the same puzzle.
Now let’s get back to where we started. 1938: early cancers can be cured.
85 years later: can we now cure all early cancers? Unfortunately not, and it’s also really, really complicated. But, do we understand better today than in 1938 why this is? Definitely.
And this takes us so much closer to where we want to be.
4. Summary
Many impressive medical discoveries have happened in cancer research in the past century.
But, the survival rate from cancer has only modestly increased.
Cancer is a very hard problem because of its evolutionary nature, always finding new ways to survive.
Useful write-up, I learned new things, thanks! It would be great if you could expand on “Cancer is a relentless evolutionary process”. Does that mean that cancer evolves like viruses do and that modern medicine could have cured people of some cancers would it not be mutating? I’m not a specialist so I lack understanding of fundamentals. Thanks!
Excellent synopsis and very excited about the new blog!!!
Why haven’t we made more progress treating cancer?
1. Many cancers are really multiple distinct subtypes of cancer co-occurring. Killing off one type can promote the other. Combining drugs is essentially impossible beyond 4 drugs because of compounding toxicities. However, exciting new work from chemical biologists like Kevan Shokat make drugs active only in specific tissues like the brain. I have high hopes for the generalization of this strategy to a lot more drugs.
2. Cancer is a genetic disease but metastasis is an epigenetic disease. And most of the deaths are coming from metastasis. Epigenetics is inherited horizontally as well as vertically and can allow cancer cells to gain properties and functions of other cell types like immune cells and neurons. These quasi-cancer cells can plug into allostatic networks which protect them from immune recognition and stimulation. Luckily neuroscience has spent the last 30 years developing the tools needed to understand rapidly evolving cell non-autonomous networks in live tissue and identified the insular circuits regulating allostasis. This work is essentially applied AI and a lot of the approaches they use can be applied to study the behavior of new AI models.
Cheers!